Using Flow Cytometry to Characterize Myeloid Derived Suppressor Cells (MDSC)
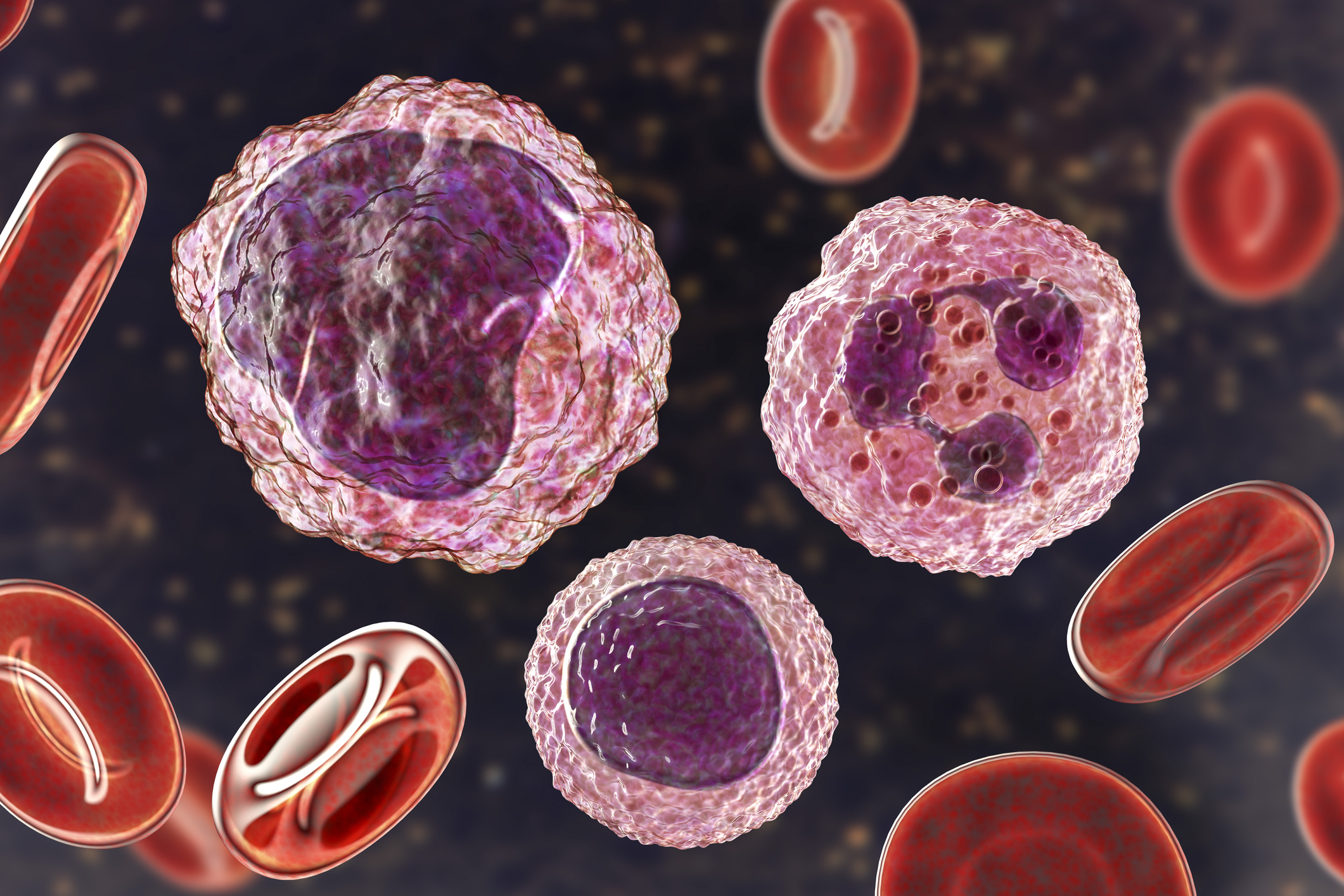
What are MDSC and why are they important? Simply put, MDSC are pathologically activated myeloid cells. The name Myeloid Derived Suppressor Cell was first coined in 2007, and in the following years MDSC have become a focus of research and drug development programs. MDSC are a heterogeneous population of myeloid-derived cells consisting of myeloid progenitors, immature macrophages, immature granulocytes, and immature dendritic cells. Notably, MDSC expand during pathological conditions such as cancer, inflammation and infection…and they are potent suppressors of T cell and NK Cell responses: Immunosuppression, through a variety of mechanisms, is their hallmark (Figure 1).

Figure 1. Mechanisms of MDSC-Mediated Immunosuppression. Graphic Courtesy of Jiseong Kim.
In healthy individuals, immature myeloid cells (IMC) differentiate into granulocytes (neutrophils), macrophages and dendritic cells. Healthy individuals do not have MDSC. Normally, myeloid cells are on the front line of host defense, however, during acute inflammation, neutrophils and monocytes expand in response to DAMPS (Damage Associated Molecular Pattern Molecules), and PAMPS (Pathogen Associated Molecular Pattern Molecules), with robust phagocytosis, respiratory burst and cytokine release. But, in pathological, chronic-inflammatory conditions such as cancer, infectious disease, autoimmune diseases or sepsis, a block in normal myeloid differentiation occurs, and the persistent stimulation of myelopoieses results in the expansion of MDSC. These cells are a mixed bag of pathologically activated myeloid cells with granulocytic and monocyte morphology, but have a different genomic profile than normal monocytes and granulocytes (Fleming, 2018, Gabrilovich, 2017).
When MDSC are activated, they produce high levels of ROS (Reactive Oxygen Species), inducible Nitrogen Oxygen Synthase 2 (iNOS), nitrogen oxide (NO), and arginase (ARG1), which further drive expansion of suppressor cells. L-arginine is the substrate for iNOS to generate NO and arginase. MDSC produce peroxynitrate (from NO and superoxide), the most powerful oxidant in the body. Peroxynitrates are found at sites of MDSC and inflammatory cell accumulation, and high levels of peroxynitrates are associated with tumor progression. The main factors involved in MDSC-mediated immune suppression are arginase (ARG1), iNOS, TGFb, IL10, PGE2 COX2, and indoleamine 2, 3-dioxygenase (IDO) (Veglia, 2018). Reactive nitrate species (RNS) nitrates tyrosine residues in proteins involved in T cell function. Together, or collectively, these suppressive factors cause the loss of the TCR zeta chain, promote nitration or nitrosylation of TCR, CD3, CD8, and CCL2, disrupt IL-2 signaling, and inhibit T cell proliferation. MDSC are thus an obstacle to immunotherapy: resistance to Immune Checkpoint blockade (ICB) treatment (anti-PD-1, anti-CTLA-4) is linked to nitrosylation of T cell surface proteins (Feng, 2018, Gabrilovich, 2017).
There is evidence linking the accumulation of immature MDSC-like cells during chronic inflammation to early stages of tumor development, and the accumulation of MDSC in lymph nodes and tumors is also associated with cancer progression and poor overall survival (Gabrilovich, 2017). Once within the tumor, MDSC evolve into potent regulators of tumor-associated immune suppression, cell invasion, and metastases, largely by contributing to the maintenance of a nasty, high-oxidative stress environment. MDSC also drive tumor progression by production of VEGF, supporting angiogenesis and the remodeling of the tumor microenvironment (TME).

Figure 2. Surface Markers Defining M-MDSC and G-MDSC. From Elliot, 2017.
Human G-MDSC are defined as Lin– HLA-DR– CD11b+ CD14– CD15+ CD33+ CD66b+
Human M-MDSC are defined as Lin– HLA-DR–* CD11b+ CD14+ CD15– CD33+ CD66b–
*HLA-DR expression separates normal monocytes from MDSC
It is now recognized that there are several phenotypes of MDSC: G-MDSC are more like neutrophils, while M-MDSCS are more like monocytes (Elliot, 2017), Figure 2. How do we identify and characterize this mixed bag of pathologically activated myeloid cells? This is where flow cytometry comes in: In the past several years, cell surface markers have been identified to discriminate between the different MDSC populations (Elliot, 2017, Veglia, 2018, Gabrilovich, 2017).
An example of flow cytometry gating is shown in Figure 3.

Figure 3. Flow Cytometry Gating Strategy for human MDSC. In the first cytogram, lineage negative/HLADR- cells are gated. From that gate, CD33+/CD11b+ cells are gated (Cytogram 2). Finally, cells are displayed as CD14 vs CD66b in Cytogram 3. M-MDSC are identified as CD14+/CD66b-. G-MDSC are identified as CD14-/CD66b+. Also, within these gated M-MDSC and G-MDSC populations it is possible to measure functional measures such as Arginase and IDO expression using flow cytometry.
The clinical significance of MDSC in cancer (and inflammatory diseases) is established. It’s recognized that MDSC are a major obstacle to immunotherapy, thus methods to selectively target MDSC and their suppressor activity are being developed (Fleming, 2018, Elliot 2017). Some approaches for inactivating MDSC include targeting PDE-5, PGE2, and COX-2 (reviewed in Gabrilovich, 2017). Multiple-other drug-development approaches to inhibit MDSC are ongoing (Fleming, 2018, listed 35 ongoing clinical trials targeting MDSC).
At FCSL, we recognize the exciting biology and increasing importance of MDSC research. We are a contract flow cytometry lab and routinely run up to 10-color flow cytometry panels in support of basic research, non-clinical and clinical studies. We are experts in flow cytometry panel design, including fit-for-purpose flow cytometry panels, Immunophenotyping, Receptor Occupancy Assay (ROA), and the inclusion of proprietary markers into established flow cytometry panels. If your research involves MDSC, please contact us for more information.
References:
Elliot, L.A., 2017, Human Tumor-infiltrating Myeloid Cells: Phenotypic and Functional Diversity, Frontiers in Immunol, Vol 8: 1-16.
Feng S., 2018, Myeloid Derived Suppressor Cells Inhibit T cell Activation through Nitrating LCK in Mouse Cancers, PNAS, 115 (40): 10094-10099.
Fleming, V., 2018, Targeting Myeloid-Derived Suppressor Cells to Bypass Tumor-Induced Immunosuppression, Front Immunol, 9 (39): 1-11.
Gabrilovich D.I., and Nagaraj S., 2009, Myeloid-Derived-Suppressor Cells as Regulators of the Immune System, Nat Rev Immunol, 9(3):162–74.
Gabrilovich D.I., 2017, Myeloid Derived Suppressor Cells, Cancer Immunol Res; 5(1): 3-7.
Veglia, F., 2018, Myeloid-Derived Suppressor Cells: Coming of Age, Nat Immunol 19(2): 108-119.
https://resources.rndsystems.com/images/site/rnd-systems-mdsc-br.pdf